Information about the Zhang Lab.
The Zhang Lab studies how signal transduction regulates cell fate determination and how normal signaling processes are compromised in disease conditions. Using live-cell imaging and optogenetic control of signal transduction, we observe and perturb signaling modules to define cellular responses. Our major goal is to gain insight into signaling mechanisms that regulate critical cellular functions such as cell proliferation, differentiation, migration, and apoptosis and to apply the insight to understanding and treating neurological disorders.
Our Principal Investigator is Kai Zhang.
Check out our website here!
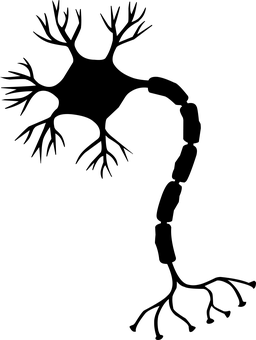
Axonal transport in neurological disorders
Neurons are the most polarized cell types, extending processes 10,000 times the size of their cell bodies. In such a polarized cell, Brownian diffusion is not sufficient to drive efficient communications across the whole neuron cell. For a small molecule to diffuse from the hand to the brain, it will take about 30 years! Instead, communication between different parts of neuronal cells requires active transport. Cargoes containing signaling molecules or newly synthesized proteins are transported along the cytoskeletal tracks of axons, very similar to the vehicle transport on highways. Defective axonal transport has been observed in neurological disorders such as Alzheimer’s disease, Parkinson’s disease, Amyotrophic Lateral Sclerosis (ALS), and Charcot-Marie-Tooth neuropathy, to name a few.
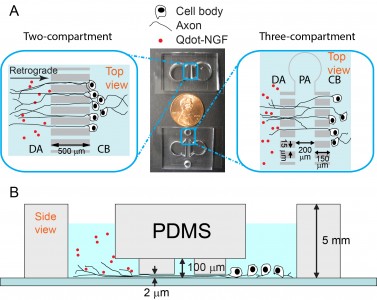
We study the neurotrophic signaling pathway that primarily regulates neuronal differentiation and survival. Our previous work has shown that axonal transport can be either slowed down or accelerated in neurological disorders (Figure 1). We use compartmentalized microfluidic devices to spatially segregate and control the chemical environment of axons and cell bodies (Figure 2). We use live-cell imaging to track the axonal transport of fluorescently labeled cargos. Our long term goal is to determine how axonal transport is affected by specific neuronal phenotypes and how manipulation of axonal transport may rescue these phenotypes.
Movie 1
Photophysics of fluorescent carbon nanodots revealed at the single-particle level
Fluorescent carbon dots are emerging nanometer-sized materials that are blessed with biocompatibility, versatile emission wavelength tuning, photostability, and economical synthesis. Recently, fluorescent carbon dots have been increasingly used in bioimaging and biosensing, although their photophysics has not been well understood. Unlike our previously investigated nanoparticles such as quantum dots, photophysics of carbon dots depends on their surface states instead of particle size. In a collaboration with Dr. Dipanjan Pan, we set out to investigate the photophysics of carbon dots at the single-particle level. We are particularly interested in determining how synthetic conditions such as temperature and precursor composition affects the fluorescent emission properties of carbon dots.
Spatiotemporal control of growth-factor signal transduction by optogenetics
Cells are constantly making decisions in response to their environments. Intracellular signal transduction transmits external stimuli into the cell interior and regulates transcription and translation. Growth factor-mediated signal transduction regulates a wide spectrum of cellular functions such as cell proliferation, differentiation, migration, and apoptosis (Figure 4). Dysregulated growth factor signaling has been observed in various diseases including cancers or neurological disorders. Intriguingly, different growth factors trigger distinct cellular functions via activation of similar downstream signaling cascades such as the mitogen-activated protein kinase (MAPK), phosphoinositide 3-kinase (PI3K)-AKT, and phospholipase C (PLC). Consequently, a central question in growth-factor mediated signal transduction is how the same set of signaling cascades elicits such diverse yet specific cellular outcomes.
Previous research has suggested that cells employ spatiotemporal regulation of their signaling cascades to convey the specificity of cell fate determination. For instance, epidermal growth factor (EGF) triggers a transient MAPK activation and induces cell proliferation; nerve growth factor (NGF) triggers a sustained MAPK activation and induces cell differentiation. Directed cell migration is driven by asymmetric subcellular activation of actin dynamics. Conventional pharmacological and genetic approaches have continuously expanded our knowledge base of signaling components involved in signaling networks. These approaches, however, lack the resolution of spatial and temporal control. A better understanding of signaling mechanisms, therefore, calls for new tools that can precisely control intracellular signaling in both space and time.
Optogenetics combines the power of light and genetics and enables precise spatial and temporal control of individual signaling cascades. Our previous work used light to control the MAPK signaling pathway and quantitatively revealed the kinetic effect of the MAPK signaling on cell differentiation (Movies 1 and 2 below).
In the long term, we aim to
- Extend optogenetic modules for activating other growth factor signaling pathways.
- Dissect how spatiotemporal regulation of growth-factor signal pathways determine cell fate.
- Develop new optogenetic tools using protein engineering, computation, and genetic screening.